Plastic strain-induced transition in silicon reveals novel transformation sequence
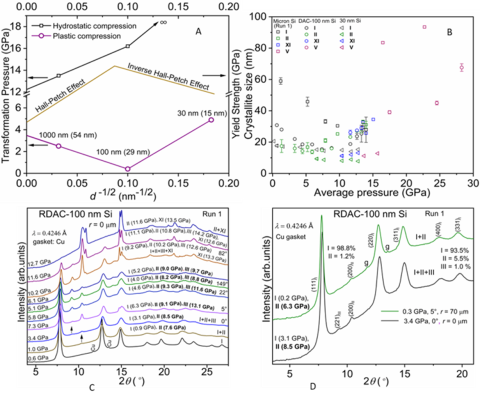
Researchers from Iowa State University and HPCAT recently performed measurements on silicon using the novel rotational diamond anvil cell and found that shear and non-hydrostatic compression results in formation of Si-II at lower pressures and at ambient temperature. This study spearheaded by long-time HPCAT user, Prof. Valery Levitas (Anson Marston Distinguished Professor in Engineering and Murray Harpole Chair in Engineering at Iowa State University), was recently published in Nature Communications.
Traditional pressure and stress-induced phase transitions (PTs) between numerous phases of Si, the most important electronic material, have been studied for decades. However, plastic strain-induced PTs are entirely different and promise numerous discoveries. It was predicted theoretically that a reduction in the grain size leads to an increase in the yield strength (Hall-Petch effect) and then to a decrease in the yield strength (inverse Hall-Petch effect). Correspondingly, the minimum pressure for strain-induced PT first reduces and then increases. This was confirmed with the appearance of Si-II and is opposite to what we observed in the hydrostatic compression experiments. Pressure in small Si-II and Si-III regions was found to be ~5-7 GPa higher than in the parent Si-I. Both results strongly support the suggested mechanism of strain-induced PTs by nucleation at the tip of the dislocation pileup. For Si powder with an average particle size of 100 nm, strain-induced PT Si-I→Si-II initiates at 0.3 GPa versus 16.2 GPa under hydrostatic conditions (i.e., pressure is reduced by a factor of 54!); for 30 nm Si, it is 4.9 GPa versus ∞, since this PT does not occur at all under pure compression.
Plastic straining also changes the sequence of PTs and leads to the coexistence of four Si phases. Based on the understanding gained from our studies, several long-standing puzzles have been resolved. Most importantly, retaining Si-II at ambient pressure and initiating the reverse Si-II→Si-I PT, have been observed. Single-phase nano Si-III has been obtained in the relatively large region of the sample after compression of 100 nm Si to 11.6 GPa and unloading within several minutes. This contrasts one of the existing techniques of producing Si-III, which requires quenching from 14 GPa and 900 K over three days. Nanocomposite of two semiconducting phases Si-I+Si-III in 100 nm Si retained after unloading from 5.2 GPa may have useful material properties.
Our results open a new direction in developing the scientific foundation for plastic strain- and defect-induced material synthesis. Retrieving the desired nanostructured pure phases or mixture of phases (nanocomposites) with optimal electronic, optical, and mechanical properties is possible with this technique. These results also challenge the current theoretical description of these phenomena. Concurrently, reduction in the PT pressures by more than an order of magnitude and their nontrivial scale dependence require serious reconsideration for other engineering problems. Thus, they are important for understanding and optimizing surface treatment (polishing, turning, etc.) of strong brittle semiconductors and ceramics, developing regimes of their ductile machining by promoting PTs to ductile phases, and for friction in NEMS/MEMS. Also, these results warn us that some Si phases may appear during surface treatment of Si wafers and friction while they are undesirable.
Details of this study can be found in the following publication - Yesudhas S., Levitas V.I., Lin F., Pandey K. K., Smith J., Unusual plastic strain-induced phase transformation phenomena in silicon, Nature Communications, 2024, Vol. 15, 7054, https://doi.org/10.1038/s41467-024-51469-5