New rules of nanostructure evolution, severe plastic deformations, and friction under high pressure revealed by in-situ study of Zr with rough diamond anvils
Recently published work on non-hydrostatic compression of strongly plastically pre-deformed Zr using new rough diamond anvils (rough-DA) and in-situ x-ray diffraction reveals new general rules of nanostructure evolution, severe plastic deformations, and friction under high pressure. The research effort was led by a long-time HPCAT user, Professor Valery I. Levitas, Anson Marston Distinguished Professor in Engineering/Murray Harpole Chair in Engineering at Iowa State University and faculty scientist at Ames National Laboratory, his postdocs Feng Lin and Sorb Yesudhas, and former postdoc Krishan K. Pandey (currently scientific officer at Bhabha Atomic Research Center, Mumbai, India). The collaborative effort with HPCAT-APS colleague Changyong Park was recently published in Materials Research Letters in the paper “In-situ study of rules of nanostructure evolution, severe plastic deformations, and friction under high pressure”.
The main difficulty in studying plasticity, structural changes, and contact friction is that they depend on five components of the plastic strain tensor and its entire path, making an unspecifiable number of combinations of independent parameters. Plastic straining causes changes in the yield strength (hardening or softening) and strain-induced anisotropy. A known method to determine pressure dependence of the yield strength based on the measured in diamond anvil cell (DAC) pressure gradient and sample thickness assumes that the friction stress reaches the yield strength in shear. However, we found that it is approximately two times lower. To resolve a problem, rough-DA, whose culet is roughly polished to increase friction, are introduced. It is demonstrated that maximum friction equal to the yield strength in shear is reached with rough-DA, which allows robustly determining pressure-dependent yield strength in compression and plastic friction. It is shown that after the α-ω phase transformation, for four different compression stages, all pressure distributions of ω-Zr are described by single function. This is possible only if the material behaves like perfectly plastic (i.e., without hardening or softening), isotropic, and independent of plastic strain and its full path. Similarly, friction stress equal to the yield strength in shear is also independent of plastic strain and its full path. The perfectly plastic state is connected to reaching a steady nanostructure, determined by in-situ synchrotron XRD in terms of crystallite (grain) size d and dislocation density, which do not change under successive plastic straining and is also independent of plastic strain and its full path, and pressure. For rough-DA in α-Zr at the beginning of α-ω transformation, crystallite size is smaller, and dislocation density is larger than those from smooth anvils, i.e., rough-DA produce a different, more refined steady nanostructure, and corresponding different surfaces of perfect plasticity with different yield strengths. This leads to the new key problem in plasticity theory: for which classes of plastic strain and its full path and maybe pressure path material behave along each of the surfaces of perfect plasticity, and for which loading classes does the material behavior jump from one surface to another? The solution to this problem will allow one to explain why different severe plastic deformation technologies lead to different steady grain sizes and dislocation densities and how to design the loading paths to reduce the grain size and increase dislocation density and strength. The obtained results suggest a more economical way to produce the desired steady nanostructure by combining severe plastic straining first at normal pressure and then at high pressure. Rough-DA intensify plastic flow, change its character and steady nanostructure.
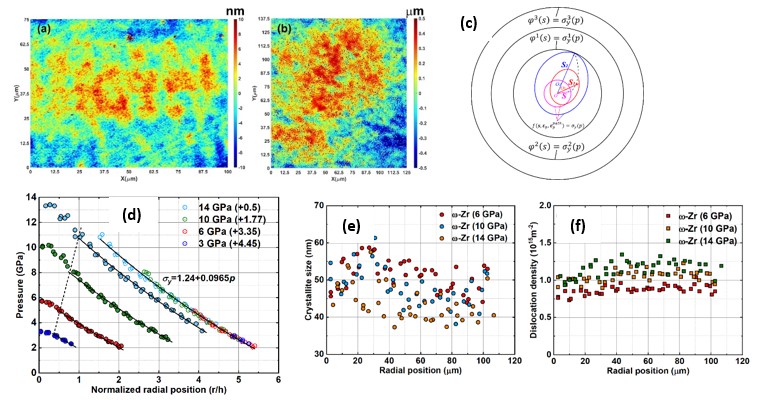
Figure: Surface asperity profile of smooth and rough anvils Multiple surfaces of perfect plasticity - (a) Traditional smooth-DA with an asperity profile range [-10 nm; 10 nm] and (b) rough-DA with a range [-500 nm; 500 nm] to maximize contact friction reaching the pressure-dependent yield strength in shear. (c) Evolving yield surface and several fixed surfaces of perfect plasticity are shown in “5D” space of deviatoric stresses at fixed pressure p. When the yield surface reaches any surface of perfect plasticity, the material deforms like perfectly plastic, isotropic, and independent of plastic strain tensor
and its entire path
. (d) Radial distributions of pressure in single-phase ω-Zr, all described by the same pressure dependence of the yield strength. Radial distribution of the crystallite size (e) and dislocation density (f) in ω-Zr for three loading steps. Since
,
, and p strongly vary with radius and increasing load, independence of the crystallite size and dislocation density of radius and load indicates that steady nanostructure in terms of crystallite size and dislocation density, which is independent of pressure,
and
, is reached.
For more, see: Lin F., Levitas V.I., Pandey K.K., Yesudhas S., and Park C. In-situ study of rules of nanostructure evolution, severe plastic deformations, and friction under high pressure. Materials Research Letters, 2023, 11, 757-763. https://doi.org/10.1080/21663831.2023.2231983